Have you ever wondered about the intricate dance of photons that underpins the very fabric of our universe? The photon, an elementary particle and a quantum of the electromagnetic field, plays a pivotal role in the study of light and its properties. This article delves into the complexities of photon energy calculations, exploring how single-photon-added coherent states transform classical light fields with just one quantum of excitation.
The photon is not merely a particle but a bridge between the quantum and classical realms. It carries electromagnetic radiation such as light and radio waves, acting as the fundamental unit of interaction in the electromagnetic spectrum. Understanding photon energy involves determining either the frequency or wavelength of light. Depending on the given parameters, the calculation process varies, yet it remains integral to advancing physics knowledge. Let us explore this fascinating subject further by examining its theoretical underpinnings and practical applications.
Attribute | Details |
---|---|
Name | Albert Einstein |
Date of Birth | 14 March 1879 |
Place of Birth | Ulm, Kingdom of Württemberg, German Empire |
Field of Expertise | Theoretical Physics |
Notable Work | Quantum Theory of Light, Theory of Relativity |
Awards | Nobel Prize in Physics (1921) |
Reference | Nobel Prize Biography |
On this day in 1908, Albert Einstein presented his groundbreaking quantum theory of light, marking a significant milestone in scientific history. His work laid the foundation for modern physics, offering profound insights into the nature of light and its behaviour at the quantum level. By introducing the concept of light quanta, later known as photons, Einstein revolutionised our understanding of electromagnetic radiation. This theory explained phenomena such as the photoelectric effect, which earned him the Nobel Prize in Physics in 1921.
In the realm of quantum optics, single-photon-added coherent states represent the simplest form of amplification for classical light fields. These states arise when a single quantum of excitation enhances the electromagnetic field. Such processes are crucial in quantum information science, enabling precise control over quantum particles and facilitating advancements in quantum computing and communication technologies. The ability to manipulate individual photons opens new avenues for research and innovation, pushing the boundaries of what is possible in the quantum domain.
Quantum analysis of light propagation in dispersive nonlinear media provides valuable insights into the behaviour of electromagnetic waves. Through quantization procedures, scientists can model the intricate interactions between light and matter within complex systems. For instance, analysing light propagation in multimode degenerate parametric amplifiers reveals how macroscopic linear and nonlinear polarizability influence wave dynamics. This formalism proves particularly effective in describing dispersive environments, enhancing our comprehension of light's quantum properties.
Photon energy calculations serve as a cornerstone for various scientific disciplines, from astrophysics to telecommunications. By determining the frequency or wavelength of light, researchers can compute photon energies using Planck's constant. Step-by-step examples guide learners through these computations, fostering a deeper appreciation for the principles governing quantum mechanics. Mastery of these techniques empowers physicists to tackle real-world challenges, driving technological progress across industries reliant on light-based technologies.
A quantum of light refers to a specific quantity of electromagnetic radiation, often equated to a single period of a sine wave. This conceptualisation simplifies the representation of light quanta, facilitating discussions about their characteristics and interactions. While seemingly abstract, this definition aligns with experimental observations, reinforcing the validity of quantum theories. Moreover, it underscores the discrete nature of energy exchanges in the microscopic world, contrasting sharply with classical continuum models.
Innovative experiments conducted by teams at the University of Ottawa exemplify the potential of quantum simulators. Utilising well-understood quantum particles like photons, researchers simulate complex systems to uncover novel phenomena. Their work highlights the versatility of quantum technologies, demonstrating their capacity to address previously insurmountable problems. As these tools continue to evolve, they promise transformative impacts on diverse fields, including materials science, cryptography, and artificial intelligence.
Historical milestones, such as Einstein's presentation of his quantum theory of light in 1908, remind us of the enduring quest for knowledge that defines humanity. Each discovery builds upon earlier achievements, creating a tapestry of understanding that enriches our collective wisdom. From deciphering the mysteries of photon behaviour to harnessing quantum effects for practical purposes, science propels society forward, unlocking possibilities once relegated to the realm of imagination.
As we delve deeper into the quantum realm, the significance of photons becomes increasingly apparent. They embody the essence of electromagnetic interactions, bridging the gap between microscopic and macroscopic scales. Through rigorous investigation and creative problem-solving, researchers unravel the secrets of light, contributing invaluable insights to the broader scientific community. Continued exploration promises exciting revelations, cementing the photon's place as a cornerstone of modern physics.
In conclusion, the study of photon energy and quantum light phenomena represents a vibrant area of inquiry, brimming with opportunities for discovery and application. By embracing the challenges inherent in this field, scientists advance our understanding of the universe while developing innovative solutions to pressing global issues. The legacy of pioneers like Albert Einstein inspires future generations to pursue excellence in research, ensuring that the pursuit of knowledge remains a cornerstone of human achievement.
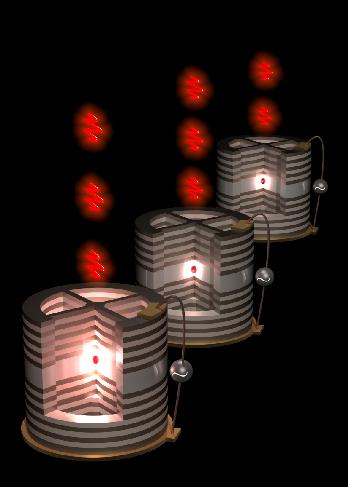

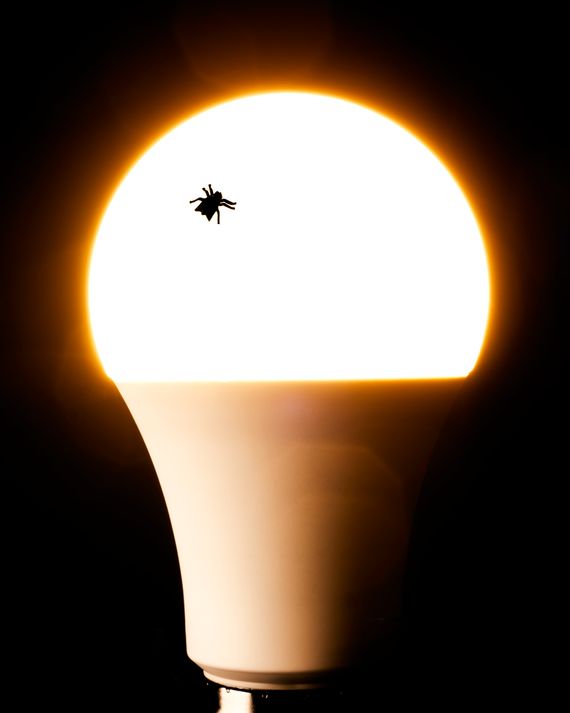
